I. INTRODUCTION
Influenza has been a human affliction since antiquity. Although the development of oseltamivir phosphate makes it possible to suppress the proliferation of influenza viruses, the efficacy of influenza vaccines remains suboptimal. The emergence of a particularly virulent strain of avian influenza poses the risk of pandemics like the Spanish influenza pandemic of 90 years ago. Some 7% of waterfowl are said to be carriers of the avian influenza virus. New virus strains continue to emerge and present ongoing threats to public health. We believe developing methods or systems for monitoring links between migratory birds and virus mutations will help make the status of communicable diseases more transparent and strengthen the planning of epidemiological preventive measures.
Recently, communication among things not previously connected to the Internet is collectively called Internet of Thinks(IoT) [1,2,3]. Managing vital data for migratory birds via digital communication would be an application of IoT in a broad sense, not limited to cases involving the use of the Internet and smartphones. Multicast video distribution and other modes of communication suggest intraflock broadcasting could be used to merge or integrate data gathered from individual birds within a flock[4,5,6]. Other key technologies supporting IoT are so-called green energy sources (solar power and conversion of kinetic energy from the flapping of wings), which power the transceivers carried by the birds [7,8,9,10]. The International Telecommunication Union (ITU) has confirmed the need to monitor migratory birds across national borders using ICT [11,12]. With data collection and wide-ranging coverage capabilities, the Argos system aboard NOAA polar orbiting satellites has been used to track migratory birds [13,14]. However, this system presents several drawbacks, including low position fix accuracy and little ability to gather other data. In response, we launched a research project based on ground wave packet communication transceivers designed to be attached to migratory birds—swans, for our purposes—and obtained biological data and positional information on these birds using bird-to-bird and bird-to-center communications.
Texas Instruments has publicly disclosed all information and data pertaining to the IC used in its CC2500 transceiver product. Disclosed on the Internet, these 3,000-odd pages of technical documents gave us the information needed to set the IF frequency bandwidth for analog circuits, modulation methods, and output levels. The availability of this information was the primary reason for choosing the CC2500. The disclosure of such extensive information—common in the United States—is rare among Japanese or Korean companies [15,16].
II. DEVELOPMENTS
We installed the TI CC2500 transceiver for packet radio communications on a bird-borne transceiver unit (circuit board = 20 g). The CC2500 accommodates transmission rates from 1,200 bps to 500 kbps, and the modulation method can be set to 2FSK, GFSK, or MSK. This report describes how we determined projected maximum communication distances after selecting the optimal modulation method, antenna gain, and circuit design.
We designed a database and implemented an RBBS (Radio Bulletin Board System). We entered biological and position information and data for each device as files into a database, with each filename based on a unique identification number and date. For bird-to-bird communications, the autonomous decentralized RBBS first exchanged data between files, then picked up files possessed by one bird, but not others. First installed in the PAX-88B packet radio communication modem by Escuela Inc. (Yokohama) in 1988, this RBBS function has been successfully applied to radio communication systems. (Nakajima Lab is the current software copyright holder.)
Communication requires establishing synchronization (for macro time and micro time) of two communicating devices. In our test unit, the synchronizing circuit of the packet communication device handled micro time synchronization, while the CPU mounted on the circuit board performed macro time synchronization based on GPS data. In cases in which GPS failed to function properly, the CPU determined the scheduled macro time based on hours of sunlight, time of sunrise, and time of sunset obtained from ambient light sensor data.
The CPU on the circuit board determines whether to rely on bird-to-bird or bird-to-center communication. For example, if the bird is located north of Hokkaido and other birds are located nearby, the transceiver selects bird-to-bird communication. The transceiver also broadcasts positional data and IDs at set intervals to provide location information. Mutual communication begins if a transceiver on another bird receives the data broadcast. In previous research on autonomous decentralized data management, we approximated their distances between each terminal based on the strength of received signal and calculated network topology such as mathematical models of ant formation. Since the CPU mounted on the circuit board performs network topology management and RBBS file management in parallel, our design called for two communication rates and two communication ranges.
The Table 1 specifies the requirements. It is thought that avian influenza requires close proximity to pass from bird to bird. Infection occurs when the virus in the intestines of a single bird is excreted and ingested by other feeding birds. Observations of swans near Lake Kutcharo and Lake Tofutsu in Hokkaido suggest a flock may span some 100–200 m in diameter. Thus, we assumed the required range of bird-to-bird radio communication to be 200 m. The range required for communication between swans and the mobile center station was assumed to be 18 km. This is two-thirds the projected line of sight from a hilltop 25 m above sea level at Cape Soya to flocks of swans observed with the unaided eye in their fall migrations from the southern tip of Sakhalin Island (Figure 1).
Demand specifications | Distance[km] | Data speed[kbps] | Communication contents |
---|---|---|---|
bird to center | 18 | 2.4 | ID confirmation |
bird to bird | 0.2 | 250-500 | file transfer |
The Figure 2 gives the internal specifications of the CC2500. The functions are described in A–D below.
The low-power S-band (2.4 GHz) packet transceiver is capable of transmitting data at rates from 1.2 kbps to 500 kbps. The FIFO serving as memory for the packets was extremely small (64-byte capacity) but nevertheless capable of handling larger packets when used in combination with the interrupt function. The modulation methods available were 2FSK, GFSK, and MSK. For structural reasons, the error correction options—FEC (Forward Error Correction) or Manchester encoding—could not be used in combination. The independent automatic repeat request (ARQ) function was disabled during the Forward Error Correction measurements. The Figure 3 is a schematic block diagram of the device.
An FEC function corrected errors generated in the transmission channel on the receiving side. Interleaving hardware on the transmitting side reordered the codes. The deinterleaver on the receiving side reversed this operation, thereby dispersing concentrated burst errors in the transmission channel.
In principle, when a code string including continuous repetitions of 000 or 11 (NRZ: Non Return to Zero) undergoes modulation, an error occurs in the determination of the number of the same codes transmitted. To eliminate this error, we implemented Manchester encoding to insert a clock component to split each bit into two to distinguish between the rising edge and trailing edge of a signal, an approach also known as biphase encoding. Generally speaking, since a failure to demodulate the clock component generates errors, Manchester encoding is suitable for data streams communicated close to the noise floor.
For codes not correctable by either FEC or CRC, the ARQ function requests a retransmission. The FEC and ARQ are mutually independent inside the CC2500 and capable of operating in hybrid mode.
The output from the packet communication device (TI’s CC2500) attached to a bird is weak: -30 dBW(0 dBm). For the transceiver unit including the antenna, we submitted an application for certification of compliance with technical standards imposed by the Japanese Ministry of Internal Affairs and Communications. Migratory birds seldom fly squarely toward the center station and often choose to fly, where possible, in the same direction as the wind. Ideally, then, beams would be emitted to the left and right of the bird. The bird-borne circuit board is extremely narrow and cannot assure sufficient gain. Nevertheless, we sought to achieve a 3 dBi gain to the right and left sides. The Figure 3 shows the balanced feed collinear antenna we designed and fabricated. For the center antenna, we used a 1 m dish antenna and measured the antenna pattern in a 10 m anechoic chamber.
III. TESTS AND RESULTS
Due to the distance between any given bird and the center and the proximity of the signal to the noise floor, slower transmission rates and Manchester encoding offer advantages over FEC. For our assessment, we connected two CC2500 boards (Figure 4), configured an attenuator between them, reduced the received power, and varied the relative C/N (signal-to-noise ratio) to determine the ideal settings for modulation method, transmission rate, and intermediate frequency (IF). FEC and ARQ were disabled for this experiment.
For the CC2500 communication control program, we used the EW430 KickStart version (JAJA173.zip) obtained at no cost from IAR Systems. This program allows the transmission of commands to the CC2500 on the target board via the PC serial port for continuous modulated wave transmission, non-modulated output, continuous receiving, and parameter setting. To confirm signal levels after the attenuator, we used a spectrum analyzer to measure error rates between the two boards, as shown in Figure 5.
Of 2FSK, GFSK, and MSK, 2FSK provided the best C/N ratios. With 2FSK selected, the frequency shift was smallest at 14.28 kHz and with Manchester encoding(+), as shown in Figure 7, while error rates were lowest at a receiving bandwidth of 101.56 kHz (Figure 6).
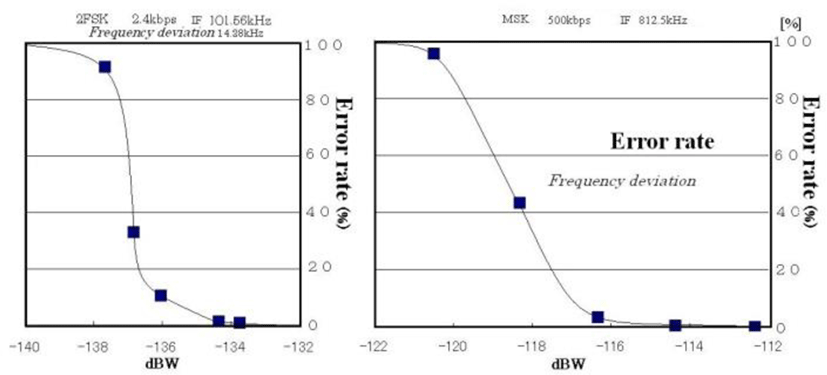
We measured the error rate for the Texas Instrument CC2500 with the modulation method set to 2FSK, frequency shift at 14.28 kHz, a transmission rate of 2.4 kbps, Manchester encoding(+), and receiving IF bandwidth of 101.56 kHz (Fig. 7). The error rate was 5% or less at -135 dBW. Changing these settings to MSK modulation method, 500 kbps transmission rate, and 812.5 kHz receiving IF bandwidth resulted in an error rate of 5% or less at -116 dBW. Since this data was communicated in packets, issuing a resend request would allow successful communication even with error rates around 20%, but at slower speed. We opted for an error rate of 5%.
The dorsally mounted antenna has lateral directionality and is powered from the left and right, with phases of the half-wavelength elements matched. This configuration is ideal for communication with adjacent birds. The antenna pattern was measured in an anechoic chamber in Toyama Prefecture measuring 10 m × 10 m × 10 m (Figure 8). The mobile center antenna is equipped with a gimbal tracking system for elevation and azimuth angle measurements to enable communication with swans in flight. The Figure 9 shows the antenna pattern of an antenna attached to a swan. The Figure 10 shows the antenna pattern of the dish antenna positioned at the center. These antenna patterns indicate maximum gains of 3 dBi and 23 dBi, respectively.
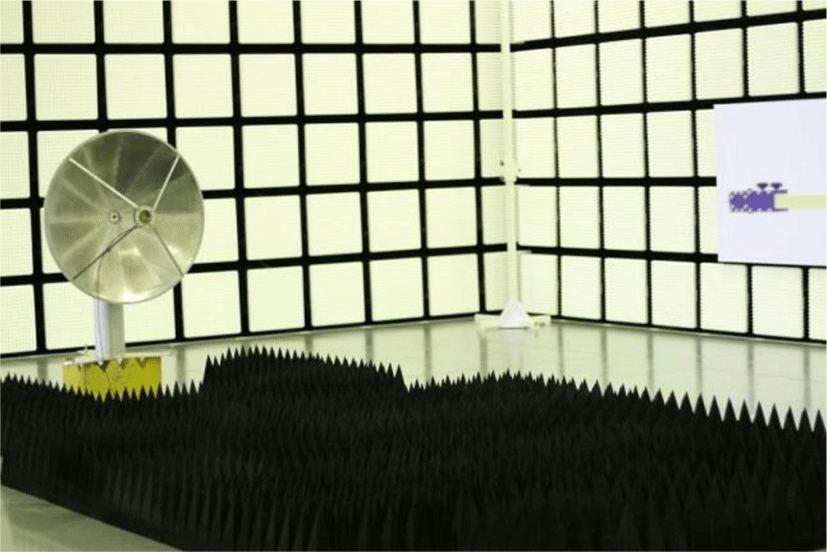
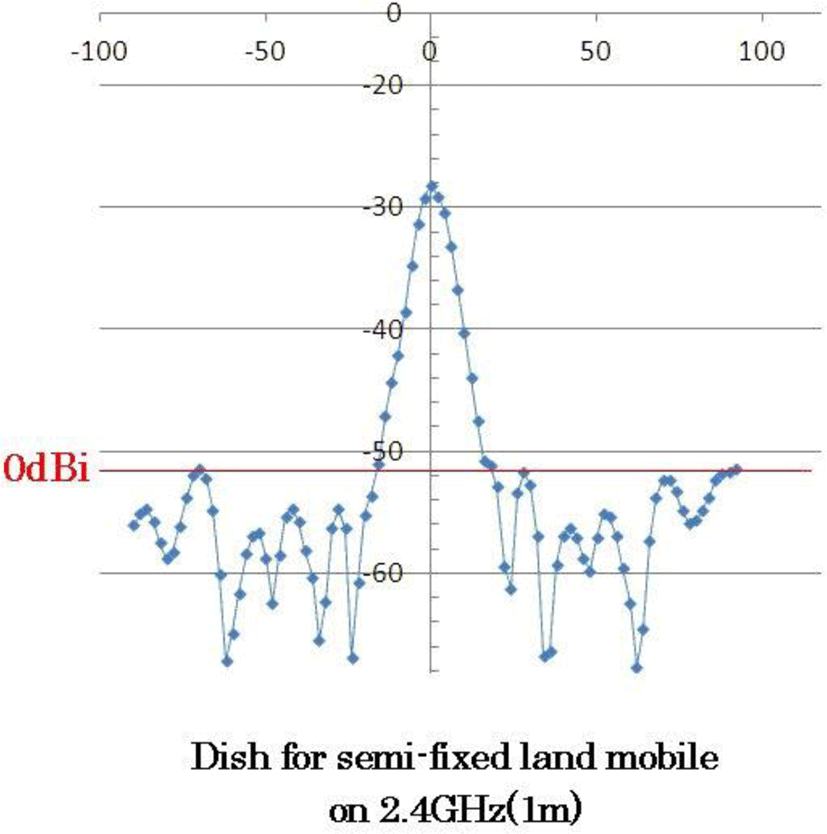
IV. CONSIDERATIONS
Based on our assessments, we determined that the ideal modulation method for bird-to-center communication over relatively long distances was 2FSK with a frequency shift at 14.28 kHz and receiving IF bandwidth of 101.56 kHz. Our assessment disregarded the effects of radio waves reflected from lake surfaces, mountains, and so forth (the propagation environment). Thus, we needed to examine signal interference using a phasing simulator and to take the results into account.
Admittedly, suggesting that MSK and GFSK are inferior to 2FSK contradicts the received wisdom. The results of our study suggest that the CC2500’s analog circuit was initially designed for use with 2FSK at the development stage, after which MSK and GFSK were later added to make the product more marketable. This underscores the benefits of thoroughly analyzing the manufacturers’ design intentions when, as in this case, designing transceivers using existing devices.
We calculated the maximum propagation distance in each communication case based on the premise of the error rate above. The results of calculations using the formula for propagation loss in free space showed 400 m at 500 kbps for bird-to-bird communication and 35 km at 2.4 kbps and in 2FSK mode for bird-to-center communication. These values met the required specifications of 200 m and 18 km.
The research consortium applied to the organization affiliated with the Japan Ministry of Internal Affairs and Communications for wireless-form authorization. After the tests performed by the official body, we obtained 28 sets of a type approval license for 2.4 GHz. In cooperation with the Yamashina Institute for Ornithology, we attempted to tag and release six or more swans (Figure 11 and 12). The transceivers attached to the back of these birds record data on respiration and cardiac beats with an angular velocity meters. They also perform store and forward data transmission to other transceivers and to the center terminal in Japan. For use in preventing avian flu, we intend to collect and analyze vital data on wild swans. Although various communication apparatus have been attached to wild birds to date[17,18,19,20,21], our system should prove remarkably capable in collecting vital data.
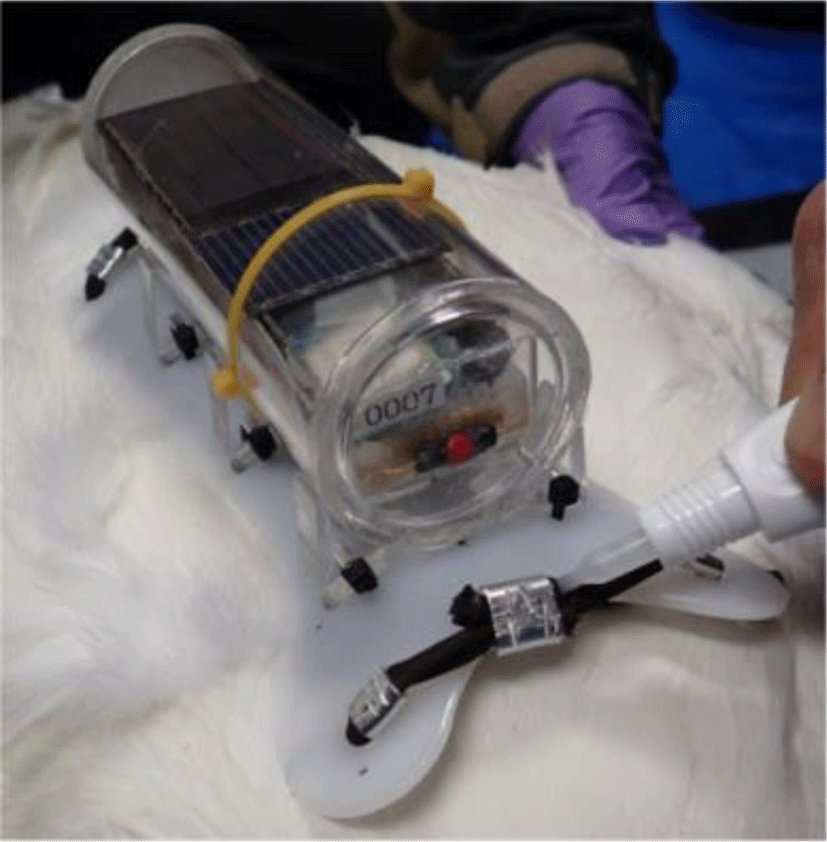
V. CONCLUSION
We designed and produced an S-band packet communication terminal to achieve bird-to-bird communication over distances of up to 200 m at 500 kbps and bird-to-center communication over distances of up to 18 km at 2.4 kbps, assuming 2FSK, 0 dBm output. We also verified the performance of this system.